An Overview of RNA Technologies
RNA technologies made headlines in 2021 when the first mRNA-based COVID-19 vaccine was approved in December 2020.
However, RNA therapy is not a new technology. RNA modalities have been under development for several decades and by Jan 2020, the U.S Food and Drug Administration (FDA) had approved a total of eleven RNA drugs, and that has increased to sixteen approved RNA therapeutics by March 2022. In addition, there are approximately another 29 RNA therapies in clinical trials as of March 2022.
The first use case of RNA as therapeutics was demonstrated in the late 1970s, Zamecnik & Stephenson demonstrated the potential of RNA antisense oligonucleotide (ASO) therapy. The duo showed that the synthesized ASOs were able to inhibit Rous sarcoma virus replication, by inhibiting viral protein translation. This was followed by the use of mRNAs for protein expression, RNAi for translation inhibition, and further developments in other formats. The development timeline for RNA modalities can be seen below.
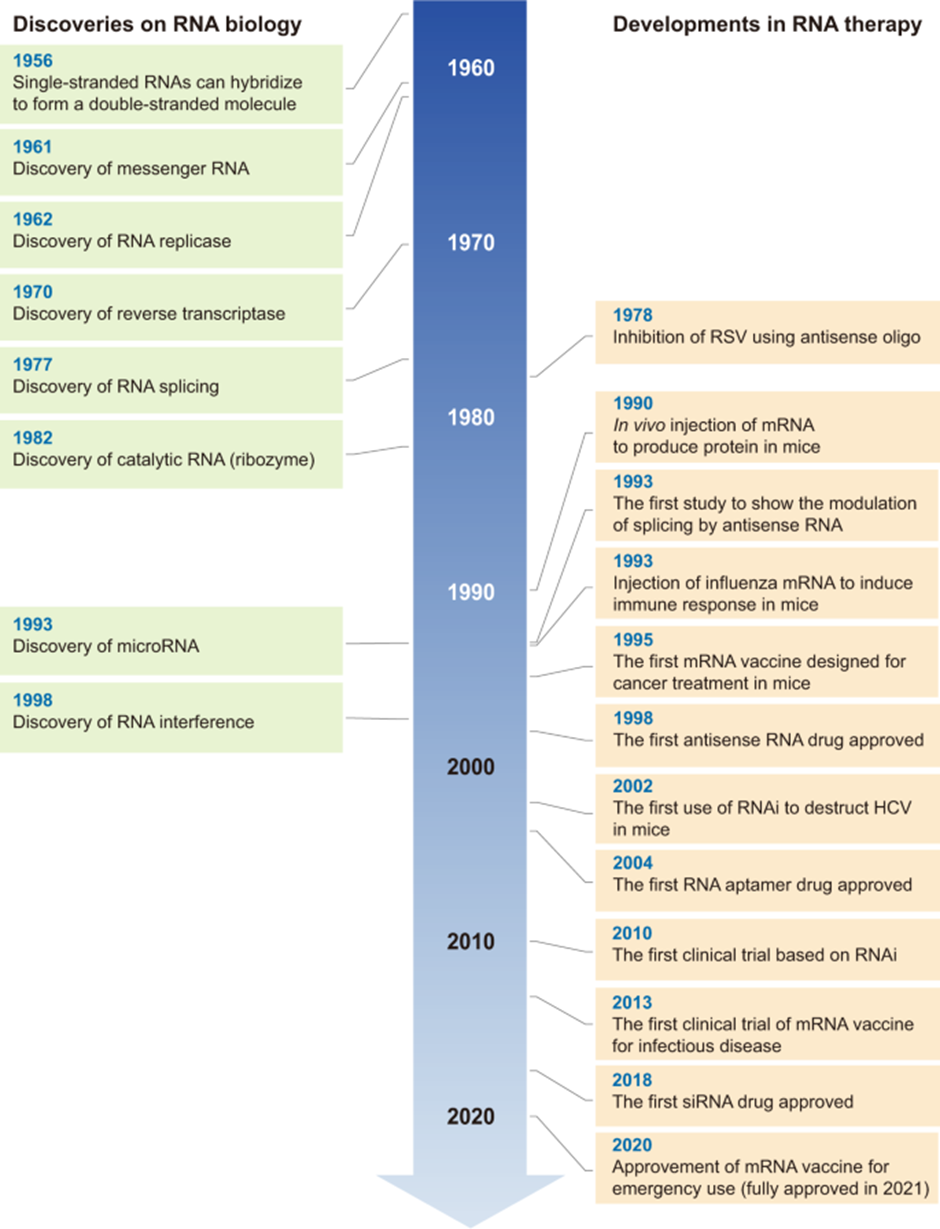
Most RNA therapies can be sorted into one of three broad categories (See image below for visuals):
1) those that target nucleic acids (either DNA or RNA), which can be further divided into two distinct types of therapies:
a. Single-stranded antisense oligonucleotides (ASOs) and,
b. Double-stranded molecules that operate through a cellular pathway known as RNA interference (RNAi)
2) those that target proteins (aptamers), and
3) those that encode proteins (mRNA).
Also, hybrid approaches that combine several RNA-based mechanisms into a single package are emerging.
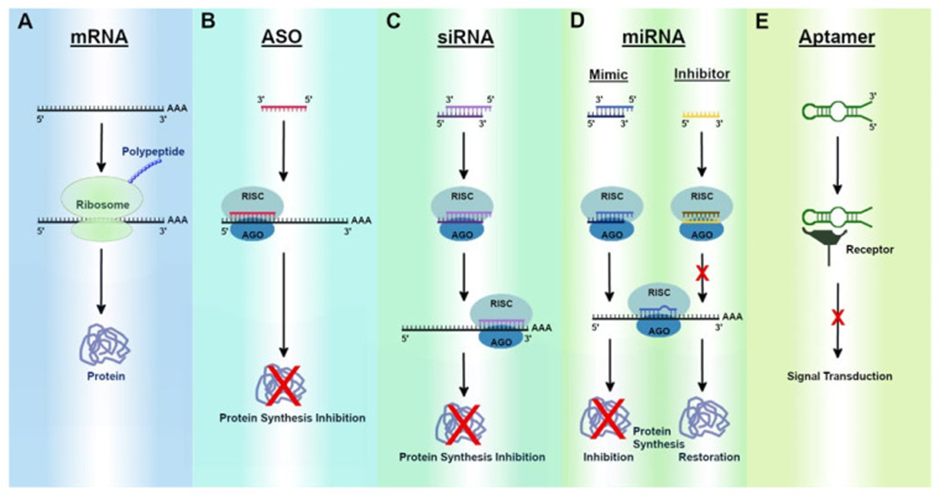
Let’s look at each of these therapeutic approaches in detail.
Antisense Oligonucleotide (ASO)
Zamecnik and Stephenson demonstrated the potential of antisense therapy by demonstrating the possibility of inhibiting protein translation with a synthetic oligonucleotide which came to be known as an ASO.
Twenty years later in 1998, the first ASO drug got its FDA approval which was developed by Ionis Pharmaceuticals and Novartis. The biotech duo developed a product named Vitravene (fomiversen) for the treatment of cytomegalovirus (CMV) retinitis. The ASO was targeted to the CMV mRNA to downregulation the expression of the IE2 protein. Following the approval of this ASO, another seven ASOs have been approved by the FDA by the end of 2021.
What are ASOs?
ASOs are short stretches of modified DNA, RNA, or RNA-DNA hybrid's made up of about 8–50 building blocks, or nucleotides. These molecules prevent mRNA translation into protein by two mechanisms:
1) Inhibiting translation by binding to the pre/mature mRNA strand can result in different outcomes or,
2) Tagging the mRNA for degradation which results in downregulation of the protein
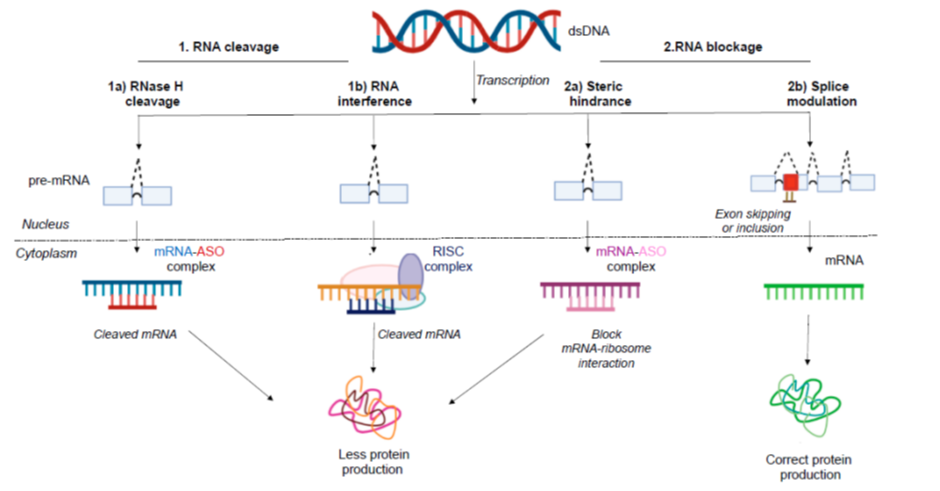
Readers can refer to our DNA article to understand the workflow from DNA to RNA to Protein.
One of the amyloidosis drugs approved in 2018, Inotersen (developed by Ionis), is an ASO. The ASO binds to the mRNA of transthyretin and thus preventing the production of the protein. The goal of this drug is thus to reduce the amount of protein production to prevent the aggregation of the TTR protein. While the efficacy of Inotersen is undoubted, it has been reported that the drug can cause serious side effects. These include low platelet counts and kidney inflammation. Because of these serious side effects, Inotersen is available in the United States only through a restricted program called the Tegsedi Risk Evaluation and Mitigation (REMS) Program.
ASOs can also alter splicing, in a process that sculpts a precursor messenger RNA into its mature form. Two such ASOs received FDA approval in 2016: Nusinersen (by Ionis), which targets a fatal inherited condition called spinal muscular atrophy, and Eteplirsen (brand name Exondys 51, by Sarepta), a treatment for Duchenne muscular dystrophy.
Nusinersen works by preventing exon-skipping thereby allowing for the production of highly stable and fully functional SMN proteins which are missing in patients with Spinal muscular atrophy.
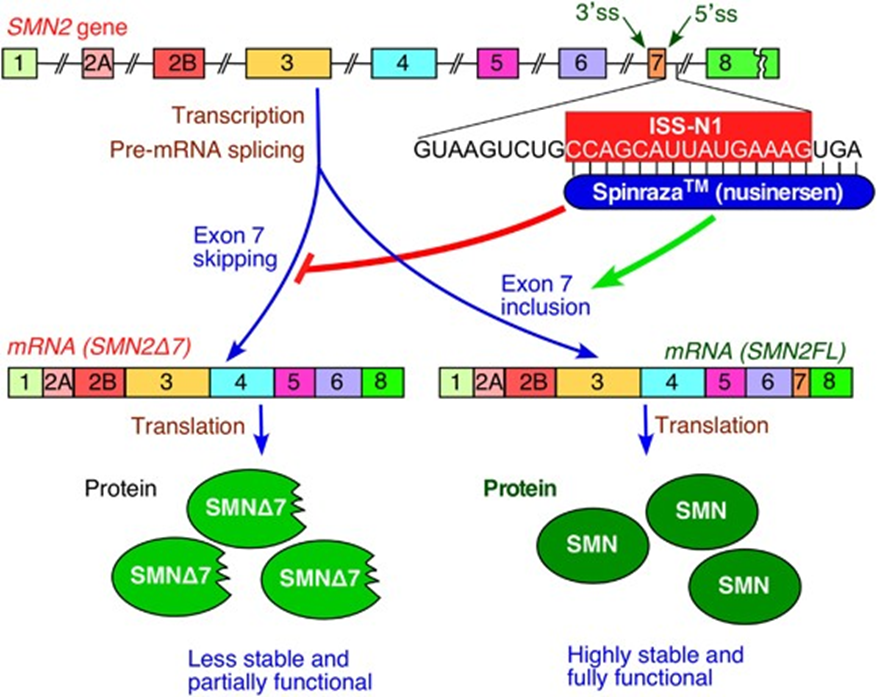
Eteplirsen on the other hand is an ‘exon’ skipping drug, which uses an ASO to block only the mutated portion of a gene from being expressed. The result is a functional protein, that lacks the mutated portion that causes pathology.
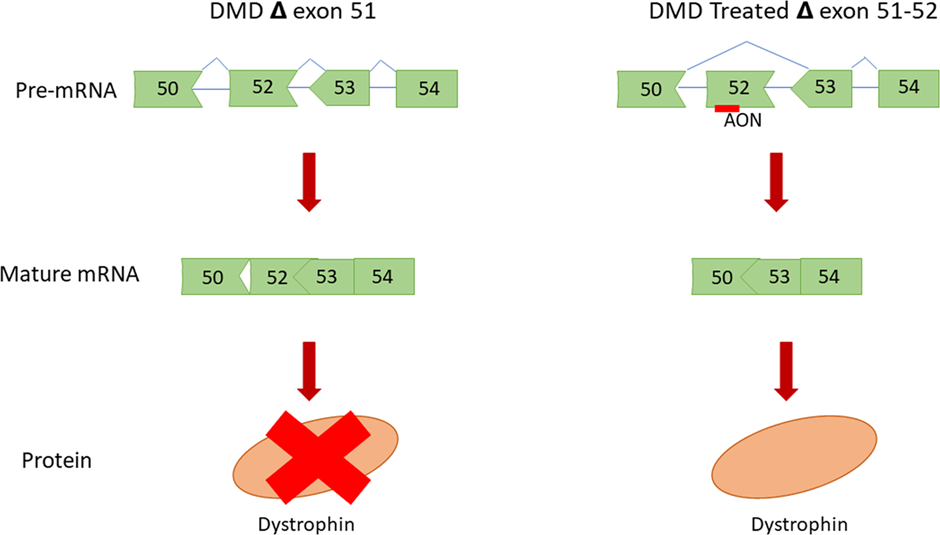
The versatility of ASOs can be seen by the broad range of diseases they can be used to treat. However, the single-stranded nature of ASOs can result in side effects in patients.
RNA Interference (RNAi)
In 1998, the same year as the first ASO therapy got approved by FDA, a team led by Andrew Fire and Craig Mello, showed that short interfering RNAs (siRNA) can suppress gene activity in Caenorhabditis elegans.
As a result of this discovery, all eyes were focused on a powerful gene-silencing technology called RNA interference (RNAi) at the start of the twenty-first century. Many companies saw the almost limitless potential of harnessing this tool to manipulate genes implicated in diseases. By 2006, Andrew Fire and Craig Mello had received the Nobel Prize in Physiology or Medicine, and large pharmaceutical companies were pouring billions of dollars into RNAi start-ups.
Because RNAi makes use of double-stranded molecules, these therapies are tougher to get into cells compared to ASOs. But fewer molecules are needed for the therapy to be effective.
RNAi involves small interfering RNAs (siRNAs), 21–23 nucleotides long, or similar molecules such as micro RNAs, to degrade mRNA and prevent them from being translated into protein. An example of siRNA therapy includes the other amyloidosis drug approved in 2018, Patisiran by Alnylam.
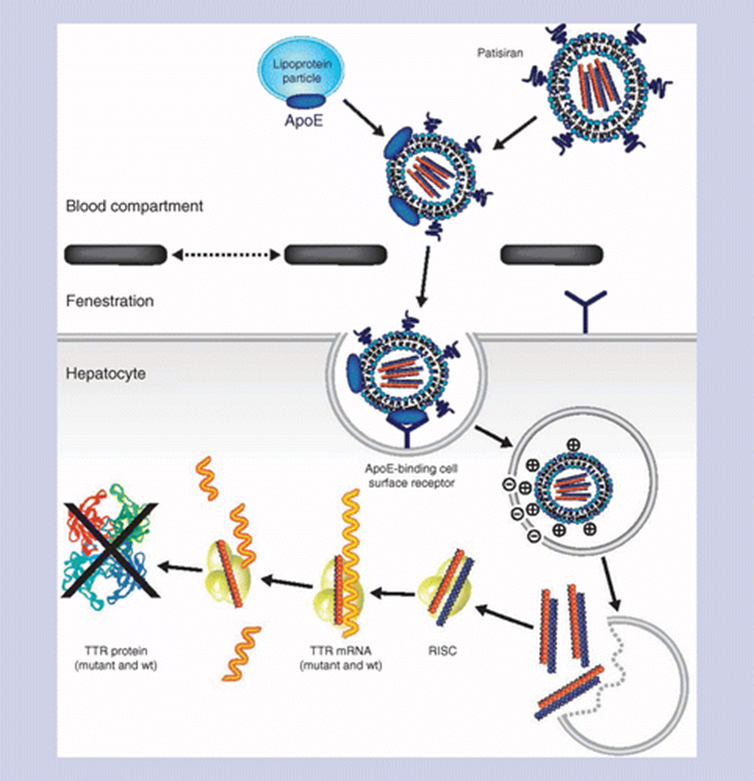
As of 2021, three siRNA-based drugs (patisiran, givosiran, and lumasiran, all belonging to Alnylam) have been approved by the US FDA, with more in Phase 3 clinical trials.
Messenger RNA (mRNA)
mRNA is coding RNA that is transcribed using genomic DNA as a template and serves to encode proteins. Despite its discovery in the 1960s the first mRNA drug/vaccine was approved only in late 2020 (COVID Vaccine).
Traditionally, mRNAs have been used for replacement therapy when diseases are caused by a lack of expression of a particular protein. Due to its main role in expressing a particular protein, mRNA therapeutics have not been widely used until recently, this is because of challenges with delivery and half-life of the mRNA strands. These are some of the key areas that scientists and companies are working to address currently. We wrote about this briefly in our previous post.
After the successful use of mRNAs as vaccines during COVID19 and the relief that side effects are mostly manageable, there are multiple trials ongoing to use mRNAs as a therapeutic. Some diseases that companies are working to address with this technology arethe expression of VEGF-A in Ischemic heart disease, expression of CFTR in Cystic Fibrosis (you can read more about this in our Vertex deep dive), and others.
Aptamers
Aptamers are single-stranded RNA, DNA, or RNA-DNA hybrids and are often classified as chemical antibodies. They often are around 20–100 base pairs long and fold into specific tertiary structures. This tertiary structure allows them to specifically bind to their respective targets which can be proteins, peptides, receptors, or other molecules. This versatility makes aptamers an attractive therapeutic option.
Aptamers mainly act through three mechanisms of action:
1. As a specific delivery molecule allowing for the targeting of tissues or cells.
2. Agonists that can functionally activate their target molecules;
3. Antagonists that can block the interaction of molecules
The first aptamer to get approval was Pegaptanib for the treatment of age-related macular degeneration (AMD) which is linked to increased VEGF expression. The aptamer worked by binding to VEGF and thereby preventing its interaction with its receptor.
Similarly, a second aptamer was approved in 2020 for the treatment of veno-occlusive disease. This is a life-threatening complication caused by chemotherapy and hematopoietic stem cell transplant (HSCT) conditioning. HSCT is increasingly being used by companies such as CRISPR therapeutics (You can read our deep dive here) and others as they look at gene-therapy-based treatments for Sickle Cell Diseases and Thalassemia.
Both approved aptamers have been shown to have minimal side effects, highlighting the promise of aptamer-based therapeutics.
Key challenges with RNA Therapeutics
Some of the key challenges that had to be addressed to make RNAs therapeutically viable as a treatment modality are as follows:
1) nucleic acids are negatively charged and do not passively cross the hydrophobic lipid barrier of the cell.
2) exogenous RNAs are degraded rapidly by RNases once they are injected into the host.
3) some exogenous RNAs cause an immune response that hampers the translation of the target protein or causes the development of a toxic cell environment
4) The short half-life of RNA.
Luckily, scientists over the past couple of decades have substantially overcome these barriers with the use of many unique delivery methods, such as nanoparticles that protect the RNA and enable cell-specific delivery of the therapeutic agent.
On the half life front, scientist initially improved the stability and half-life through a RNA modification called pseudouridine, whish replaces uridine. It is demonstrated that pseudouridine can enhance RNA stability also decrease anti-RNA immune response. As further improvements in half-life are required scientists are innovating to further improve the half-life of RNA therapeutics specifically mRNA therapeutics, read more here.
Wrapping it up
RNA therapeutics have been around for a long time, but their claim to fame came in late 2020 as the technology was essential in helping to control the pandemic. A total of four key RNA technologies exist at the moment which can be used for various purposes. We believe that RNA-based treatment modalities are still in their infancy and should continue to see strong growth in the years ahead.
If you reached here, it probably means you enjoyed reading the post. We suggest you hit the subscribe button so you do not miss out on any of our future posts. Click here to Subscribe now.
Disclaimer: All opinions shared in this article are the opinions of the authors and do not constitute financial advice or recommendations to buy or sell. Please consult a financial advisor before you make any financial decisions. The authors do not hold positions in any of the mentioned securities.
Comments ()